2023年诺贝尔生理学或医学奖得主(图片来源:诺奖官网)
北京时间10月2日下午5点45分,瑞典皇家科学院宣布将2023年诺贝尔生理学或医学奖授予:Katalin Karikó and Drew Weissman。
获奖理由
2023年诺贝尔生理学或医学奖授予“发现了核苷碱基修饰,使得能够开发针对COVID-19的有效 mRNA 疫苗成为可能”(for their discoveries concerning nucleoside base modifications that enabled the development of effective mRNA vaccines against COVID-19)。
获奖理由
NOBEL PRIZEThe discoveries by the two Nobel Laureates were critical for developing effective mRNA vaccines against COVID-19 during the pandemic that began in early 2020. Through their groundbreaking findings, which have fundamentally changed our understanding of how mRNA interacts with our immune system, the laureates contributed to the unprecedented rate of vaccine development during one of the greatest threats to human health in modern times.
Vaccines before the pandemicVaccination stimulates the formation of an immune response to a particular pathogen. This gives the body a head start in the fight against disease in the event of a later exposure. Vaccines based on killed or weakened viruses have long been available, exemplified by the vaccines against polio, measles, and yellow fever. In 1951, Max Theiler was awarded the Nobel Prize in Physiology or Medicine for developing the yellow fever vaccine.
Thanks to the progress in molecular biology in recent decades, vaccines based on individual viral components, rather than whole viruses, have been developed. Parts of the viral genetic code, usually encoding proteins found on the virus surface, are used to make proteins that stimulate the formation of virus-blocking antibodies. Examples are the vaccines against the hepatitis B virus and human papillomavirus. Alternatively, parts of the viral genetic code can be moved to a harmless carrier virus, a “vector.” This method is used in vaccines against the Ebola virus. When vector vaccines are injected, the selected viral protein is produced in our cells, stimulating an immune response against the targeted virus.
Producing whole virus-, protein- and vector-based vaccines requires large-scale cell culture. This resource-intensive process limits the possibilities for rapid vaccine production in response to outbreaks and pandemics. Therefore, researchers have long attempted to develop vaccine technologies independent of cell culture, but this proved challenging.
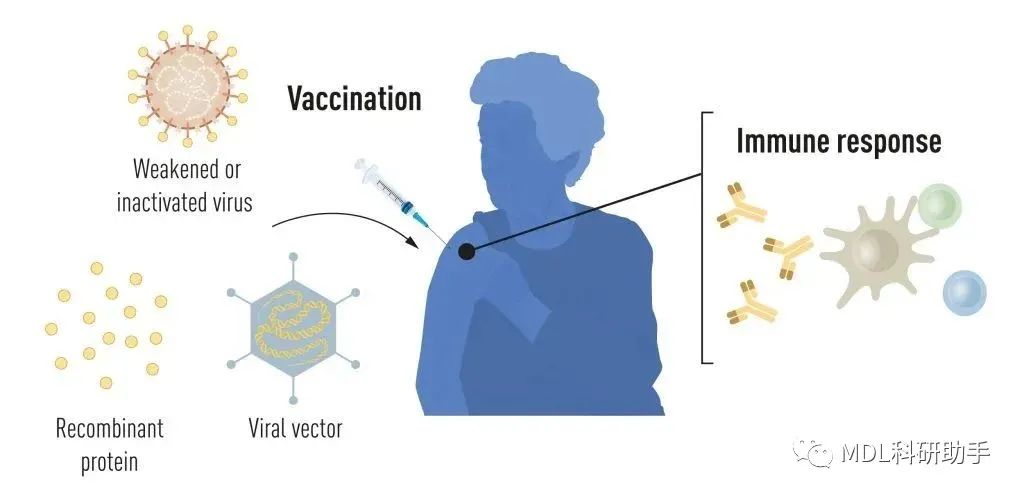
mRNA vaccines: A promising ideaIn our cells, genetic information encoded in DNA is transferred to messenger RNA (mRNA), which is used as a template for protein production. During the 1980s, efficient methods for producing mRNA without cell culture were introduced, called in vitro transcription. This decisive step accelerated the development of molecular biology applications in several fields. Ideas of using mRNA technologies for vaccine and therapeutic purposes also took off, but roadblocks lay ahead. In vitro transcribed mRNA was considered unstable and challenging to deliver, requiring the development of sophisticated carrier lipid systems to encapsulate the mRNA. Moreover, in vitro-produced mRNA gave rise to inflammatory reactions. Enthusiasm for developing the mRNA technology for clinical purposes was, therefore, initially limited.
These obstacles did not discourage the Hungarian biochemist Katalin Karikó, who was devoted to developing methods to use mRNA for therapy. During the early 1990s, when she was an assistant professor at the University of Pennsylvania, she remained true to her vision of realizing mRNA as a therapeutic despite encountering difficulties in convincing research funders of the significance of her project. A new colleague of Karikó at her university was the immunologist Drew Weissman. He was interested in dendritic cells, which have important functions in immune surveillance and the activation of vaccine-induced immune responses. Spurred by new ideas, a fruitful collaboration between the two soon began, focusing on how different RNA types interact with the immune system.
The breakthroughKarikó and Weissman noticed that dendritic cells recognize in vitro transcribed mRNA as a foreign substance, which leads to their activation and the release of inflammatory signaling molecules. They wondered why the in vitro transcribed mRNA was recognized as foreign while mRNA from mammalian cells did not give rise to the same reaction. Karikó and Weissman realized that some critical properties must distinguish the different types of mRNA.
RNA contains four bases, abbreviated A, U, G, and C, corresponding to A, T, G, and C in DNA, the letters of the genetic code. Karikó and Weissman knew that bases in RNA from mammalian cells are frequently chemically modified, while in vitro transcribed mRNA is not. They wondered if the absence of altered bases in the in vitro transcribed RNA could explain the unwanted inflammatory reaction. To investigate this, they produced different variants of mRNA, each with unique chemical alterations in their bases, which they delivered to dendritic cells. The results were striking: The inflammatory response was almost abolished when base modifications were included in the mRNA. This was a paradigm change in our understanding of how cells recognize and respond to different forms of mRNA. Karikó and Weissman immediately understood that their discovery had profound significance for using mRNA as therapy. These seminal results were published in 2005, fifteen years before the COVID-19 pandemic.
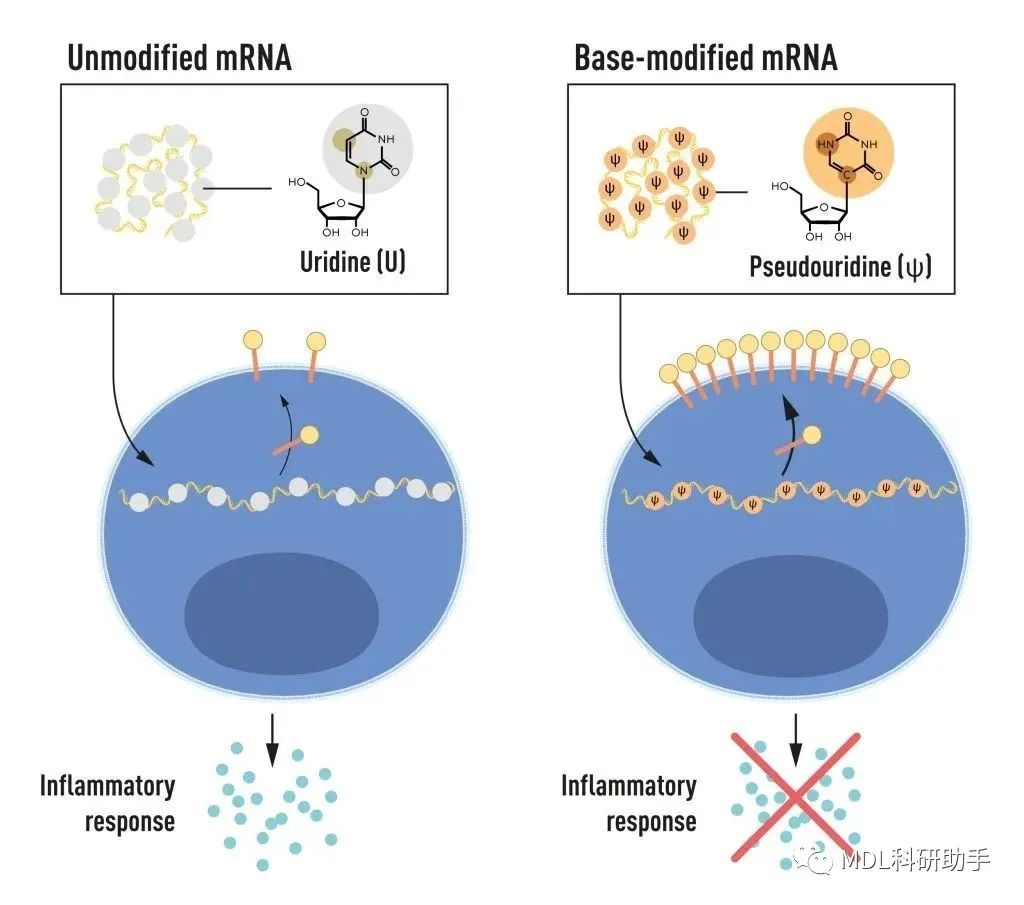
In further studies published in 2008 and 2010, Karikó and Weissman showed that the delivery of mRNA generated with base modifications markedly increased protein production compared to unmodified mRNA. The effect was due to the reduced activation of an enzyme that regulates protein production. Through their discoveries that base modifications both reduced inflammatory responses and increased protein production, Karikó and Weissman had eliminated critical obstacles on the way to clinical applications of mRNA.
mRNA vaccines realized their potentialInterest in mRNA technology began to pick up, and in 2010, several companies were working on developing the method. Vaccines against Zika virus and MERS-CoV were pursued; the latter is closely related to SARS-CoV-2. After the outbreak of the COVID-19 pandemic, two base-modified mRNA vaccines encoding the SARS-CoV-2 surface protein were developed at record speed. Protective effects of around 95% were reported, and both vaccines were approved as early as December 2020.
The impressive flexibility and speed with which mRNA vaccines can be developed pave the way for using the new platform also for vaccines against other infectious diseases. In the future, the technology may also be used to deliver therapeutic proteins and treat some cancer types.
Several other vaccines against SARS-CoV-2, based on different methodologies, were also rapidly introduced, and together, more than 13 billion COVID-19 vaccine doses have been given globally. The vaccines have saved millions of lives and prevented severe disease in many more, allowing societies to open and return to normal conditions. Through their fundamental discoveries of the importance of base modifications in mRNA, this year’s Nobel laureates critically contributed to this transformative development during one of the biggest health crises of our time.
获奖人详细信息
卡塔林·卡里科(Katalin Karikó), 卡塔林·卡里科1955年出生于匈牙利绍尔诺克。1982年,她从塞格德大学获得博士学位,并在塞格德的匈牙利科学院进行博士后研究,直到1985年。随后,她在费城坦普尔大学和贝塞斯达健康科学大学进行了博士后研究。1989年,她被任命为宾夕法尼亚大学助理教授,一直任职到2013年。之后,她成为BioNTech RNA Pharmaceuticals的副总裁,后来成为高级副总裁。自2021年以来,她一直是塞格德大学的教授和宾夕法尼亚大学佩雷尔曼医学院的兼职教授。
德鲁 · 魏斯曼(Drew Weissman), 1959年出生于美国马萨诸塞州的列克星敦。1987年,他在波士顿大学获得了博士学位和博士学位。他在哈佛医学院贝斯以色列女执事医疗中心进行临床培训,在国立卫生研究院进行博士后研究。1997年,韦斯曼在医学佩雷尔曼学院宾夕法尼亚大学成立了自己的研究小组。他是罗伯茨家庭疫苗研究教授和宾夕法尼亚大学 RNA 创新研究所所长。[color=rgba(0, 0, 0, 0.9)]来源:大健康凯歌
|